Introduction
Mercury-containing dental amalgam restorations are being replaced with dental composites as the restorative materials of choice mainly because of the inherent esthetic appeal of the latter and the long-standing controversy related to the toxicity of the former[1]. Composites are mixture of two materials in which one of the materials, called the reinforcing phase, is in the form of fibers, sheets, or particles, and are embedded in the other materials called the matrix phase. The reinforcing material and the matrix material can be metal, ceramic, or polymer. Composites are used because overall properties of the composites are superior to those of the individual components[2]. The fact that dental composite materials continue to improve in strength, abrasion resistance, ease of application, translucency, and polishability rapidly increased their use in the first decade after being introduced and continues to increase their popularity[3],[4].
History
Polymethyl methacrylate (PMMA) resins replaced the silicate cements during the late 1940’s and the early 1950’s. The advantages of these acrylic resins include tooth like appearance, insolubility in oral fluids, ease of manipulation and less expensive. However, these materials could not accomplish the requirements of restorative materials as they shrunk severely during the polymerization process; which lead to marginal leakage, had poor wear resistance, high coefficient of thermal expansion, and high water sorption. To overcome these problems, quartz powder was been added to form a composite structure. Addition of inert filler particles reduced the thermal expansion, polymerization shrinkage and water sorption of acrylic resins but increased the physical and mechanical properties. Physical and mechanical properties are mainly influenced the volume fraction of the inert fillers. These filler particles occupy the space but do not take part in the reaction[5],[6]. Another problem in the early composites was lack of proper bonding between resin and the filler particles. A major advancement was made when Dr. L. Bowen (1962) developed a new type of composite. He invented a new dimethacrylate resin, such as Bisphenol A Glycidyl methacrylate (Bis-GMA) and an organo silane coupling agent, which provides a bond between filler particles and the resin matrix[5].
Overview of Resin Composite Formulation
Dental resin composites typically contain a mixture of soft, organic resin matrix (polymer) and hard, inorganic filler particles (ceramic). The resin matrix consists of monomers, an initiator-activator system, stabilizers and pigments. The inorganic filler consists of particles such as glass, quartz and colloidal silica. The resin matrix and fillers are bonded together with the help of coupling agent. The performance of resin composites is dependent upon these basic components[5].
Resin Matrix
The resin is the chemically active component of the composite. It is initially a fluid monomer, but is converted into a rigid polymer by a radical addition reaction[7]. The most commonly used monomer for both anterior and posterior resin is Bis-GMA[8], which is derived from the reaction of bisphenol-A and glycidyl methacrylate. This resin is commonly referred to as Bowen’s resin, after its inventor. Other monomers are urethane dimethacrylate (UEDMA), and triethylene glycol dimethacrylate (TEGDMA)[5]. Bis-GMA is extremely viscous at room temperature due to hydrogen bonding by hydroxyl groups. The viscosity of Bis-GMA can be reduced by mixing with diluents and facilitate the addition of fillers. These diluents are low molecular weight dimethacrylate monomers such as triethylene glycol dimethacrylate (TEGDMA). Addition of the diluents allows greater degree of conversion and more extensive cross-linking to occur between chains – providing a matrix that is more resistant to solvents[5],[9]. Chemical formulas of various types of monomer systems used in composites are described in Table 1.
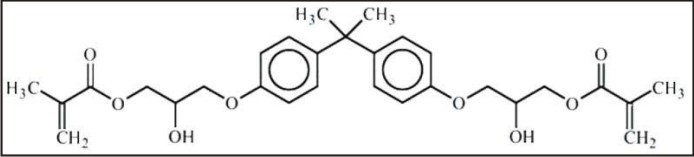 | Table 1: (i)Bis Phenol A – GlycidylDimethacrylate
 |
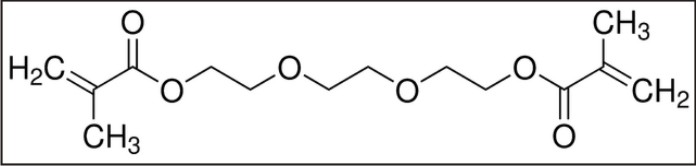 | Table 1: (ii)Triethylene Glycol Dimethacrylate (TEGDMA)
 |
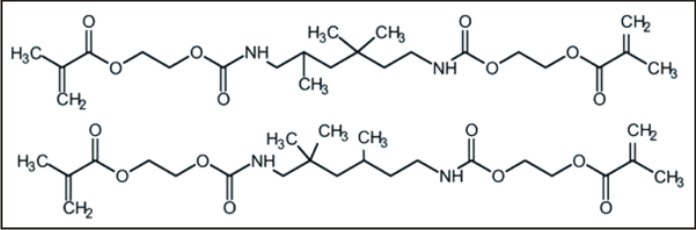 | Table 1: (iii) Urethane Dimethacrylate (UDMA)
 |
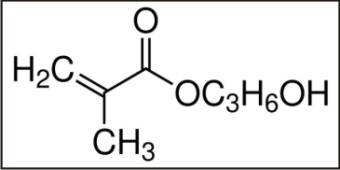 | Table 1: (iv)Hydroxypropyl Methacrylate
 |
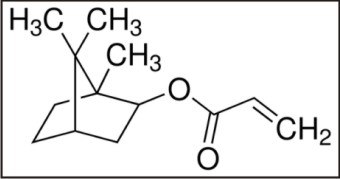 | Table 1: (v)Isobornyl Acrylate
 |
Increased conversion and cross-linking increases the polymerization shrinkage[10]. Resin composites undergo volumetric shrinkage of 1.9 to 7.1 % [11],[12].The shrinkage in the resin matrix results from the conversion of weak intermolecular attractions to primary covalent bonds[13]. BisGMA/TEGDMA resins also exhibit a high shrinkage stress which contributes to premature failure or reduced overall performance of the restoration[14],[15]. Polymerization shrinkage and the resultant stress can leads to marginal leakage. The primary goal of resin composite restorative material research is the elimination of contraction stress. Low or non-shrinking monomers can reduce this polymerization shrinkage. Hence, many alternative diluents to TEGDMA have been investigated to minimize shrinkage stress[16],[17]. Alternatives to TEGDMA are monomethacrylates and acrylates such as hydroxypropyl methacrylate and isobornyl acrylate. All these alternatives have shown reduced volumetric shrinkage and increased double bond conversions[18]. However, the reaction rate is significantly lower for these traditional functional compounds compared with that available from dimethacrylates. Polymerization shrinkage can also be reduced by increasing the concentration of filler particles since the overall shrinkage depends on the amount of polymer matrix present[5]. Bicyclic cyclopropyl-acrylates are new low viscous liquid monomers, which undergo a radical ring-opening polymerization under formation of a 1,5-substituted ring-opened unit, and are more reactive. They show lower volume shrinkage during their polymerization compared to methacrylate[19].
Filler Systems
Fillers are the inorganic or organic particles, which are added to improve mechanical properties, such as tensile and compressive strength, modulus of elasticity, abrasion resistance, radiopacity, esthetics and handling. Addition of fillers can also reduce water sorption of the resin, polymerization shrinkage, and co-efficient of thermal expansion[5]. Most current resin composites have filler loaded from 50 - 86 % by weight and 35 to 71 % by volume[20]. Filler percentage is best expressed by volume instead of weight because the mechanical properties of composites are mainly dictated by their filler volume fraction[21]. The type of filler directly influences radiopacity which is typically accomplished through the inclusion of elements of high atomic number. Barium and strontium are the most common elements used in filler particles to increase radiopacity[22]. Various classification systems for resin composites have developed over the years based on particle size[5]. The traditional system includes traditional, small particle, microfilled, hybrid, and nano filler particles[23].
Coupling Agents
Early composites lacked proper bonding between resin matrix and filler particles that resulted in decrease of mechanical properties over a period of time. It is important that the filler particle bonds to the resin matrix via a coupling agent to improve mechanical and physical properties. The most commonly used coupling agent is an organosilane such as gamma methacryloxy propyl trimethoxy silane (Table 2). The silane reduces hydrolytic breakdown and allows stress transfer between the filler and the matrix. The silane agent is a bifunctional molecule with a methacrylate group on one end and a silanol group on the other. The methacrylate end undergoes addition polymerization with the composite resin and the silanol end bonds to the hydroxyl groups on the filler particle via a condensation reaction[5].
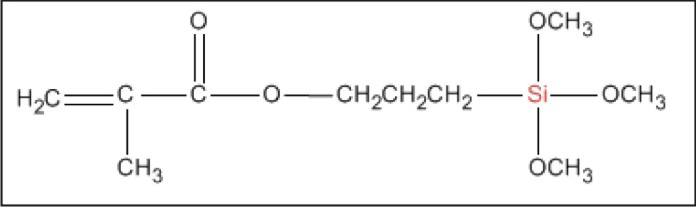 | Table 2: Gamma Methacryloxy Propyl Trimethoxy Silane Coupling agent
 |
Types of Composites
Composite resins have been classified in different ways, depending on their composition, to make it easier for dentists to identify and use them for therapeutic purposes. The most popular classification is based on filler particle size given by Lutz and Phillips. According to this classification composite resins are divided into macro filler composites, micro filler composites and hybrid composites (fillers of different sizes) [23], [24].
Conventional/traditional/Macro-filled composites
Commonly used filler is crystalline quartz, which has got excellent optical properties, and chemical inertness[3],[5]. These traditional quartz particles were produced by grinding or milling with a particle size of around 8-12 microns[5]. It is extremely hard, difficult to grind, and polish, has the potential to abrade opposing tooth structure and the chances of wearing the softer polymer leads exposing the hard quartz particle, which results in rough surface[3], [5].
Micro-filled Composites
Microfills were developed to provide better esthetics and polishability[3]. These tiny particles of silica are only 0.04 microns in diameter and are produced by a pyrolytic process. A smoother surface can be produced due to the smaller size of the silica particles[5]. These filler particles have large surface area, which requires more resin matrix to wet the surface. This results in extremely high viscosity and that limits the percentage filler content possible. In order to maximize filler loading and minimize viscosity, the use of pre-polymerized resin and micro-filler is used. The heavily filled polymerized resin is ground into 30-65 micron particles and mixed with more resin and micro-filler to provide a composite that is filled 30 to 50% by volume. However, mechanical properties such as strength and stiffness are generally inferior to larger quartz or glass filled composites because of the lower filler content, which often limits their use to non-stress-bearing areas[3]. Also, microfills are typically radiolucent which also limits their application to anterior areas.
Hybrid Composites
The most common filler today is barium glass with average particle size of 0.6 to 1.0 microns. A small amount of micro-filler is added to improve handling characteristics and reduce stickiness[3]. To incorporate a maximum amount of filler into a resin matrix, a distribution of particle sizes is necessary. These so called hybrids are potentially superior because increased filler loading improves the stress transfer between particles in the composite[5]. The current trend is to maximize filler loading and minimize filler size as with the microhybrids[3]. Other classification systems have been developed over the years due to the difficulty in categorizing the newer complicated formulations of resin composites based on a wide range of filler sizes, loading and compositions. Willems proposed a system based on volume fraction in 1992[25]. A classification system by Bayne and others, based on particle size, was introduced in 1994. The various groups included megafill – 0.5 to 2 millimeters; macrofill – 10 to 100 microns; midifill – 1 to 10 microns; minifill – 0.1 to 1.0 microns; microfill – 0.01 to 0.1 microns and nanofill – 0.005 to 0.01 microns. Most new systems are minifill hybrids with a trend toward nanofillers. Nanofilled resin composites utilize nanometer-sized particles throughout the resin matrix. Nanohybrids combine nanometer-sized particles with more conventional filler technology[26].
Posterior Composites
Recent advances in resin adhesives and restorative materials, as well as an increased demand for esthetics, have stimulated a great increase in the use of resin-based composite in posterior teeth. Properly placed composite restorations provide an excellent alternative to traditional posterior restorations. These materials have improved strength, wear resistance, handling and esthetics[20]. They reduced marginal leakage due to the availability of wide variety of dentin adhesive systems, which produce reliable micromechanical retention between composite and tooth structure[27]. They allow for a more conservative cavity preparation, preserving valuable tooth structure[28]. Manufacturers have increased the filler content and reduced the average filler particle size to produce resin-based composite materials for Class II posterior restorations, which need adequate strength and wear resistance to withstand the mastication forces to which they are subjected[29]. The disadvantages of posterior resin-based composites include, more technique-sensitive than amalgam, requiring more time for placement because they necessitate rubber dam isolation for field control and incremental build-up[20]. In larger Class II cavity preparations, it is more difficult to obtain proper contour and achieve adequate proximal contact with conventional composite than with amalgam, because conventional composite is not packable[30]. To improve ease of manipulation, the ideal resin-based composite should have a viscosity stiff enough to facilitate placement without adhering to the condensing instrument[31]. The new packable resin-based composites reportedly offer these advantages in handling[32]. Incremental build-up of resin-based composite materials has been advocated to minimize polymerization shrinkage and to facilitate adequate polymerization of composite material when preparation depths are greater than 2 millimeters[33], [34]. Uncured resin at the base of the restoration or gaps at the composite-tooth interface resulting from polymerization shrinkage can cause microleakage, with resultant pulpal sensitivity, staining and recurrent caries[35],[36],[37]. An incremental build-up technique can significantly increase restorative time.
Packable Composites
Manufacturers have developed “condensable” or “packable” resin composites marketed as amalgam alternatives. “Packable” is a more appropriate term because they are packed rather than condensed. In an attempt to make them an alternative to amalgam, some of the resins are packaged in blister packs that differ by spill size. Compared with hybrid composites, they are characterized by a higher filler load, an improved filler technology, and modifications in the organic matrices and improved handling properties. An application technique similar to that used with amalgam can be used for the placement. The use of metal matrix bands and wooden wedges allows for easier establishing of inter-proximal contacts. Their ability to be bulk cured results in a more cost-effective treatment by reducing the time needed to place a restoration. These high-viscosity resin-based composites are indicated for use primarily in cavities in load-bearing surfaces of permanent posterior teeth. Besides an improvement in handling, packable composites were expected to exhibit excellent mechanical and physical properties owing to their high filler load[32],[38] and have minimal polymerization shrinkage and an increased depth of cure, of up to 5 mm[39]. The new packable composites are reported to withstand greater occlusal forces and are recommended for use in situations in which amalgam previously was the restoration of choice[32].
Ormocer
Recently, a new organically modified ceramic packable restorative material has been developed and it is also called as ormocer. The ormocer composite consists of inorganic-organic copolymers and inorganic silanated filler particles. The newly designed inorganic-organic copolymers are synthesized in a “sol-gel,” process from multifunctional urethane and thioether(meth)acrylate alkoxy silanes[32],[40],[41],[42],[43]. The alkoxysilyl groups of the silane allow the formation of an inorganic Si-O-Si network by hydrolysis and polyconden sation reactions, and the (meth) acrylate groups are available for photochemically induced organic polymerization. After incorporation of filler particles, the ormocer composites can be manipulated by the dentist as in conventional resin-based composite material. Packable composites and the packable ormocer both have less polymerization shrinkage and can be bulk-cured[32].
Ion-Releasing Composite
An ion-releasing composite (Ariston pHc, IvoclarVivadent, Schaan, Liechtenstein) was introduced in 1998. This composite material releases fluoride, hydroxyl and calcium ions in dependence on the pH value immediately adjacent to the restorative material. With a decreasing pH value, due to active microorganisms in dental plaque, the release rate of the functional ions increases and vice versa. This phenomenon is based on newly developed alkaline glass filler and is expected to reduce the formation of secondary caries at restoration margins owing to an inhibition of bacterial growth, a reduction in demineralization and a buffering of acids produced by cariogenic microorganisms[32].
Indirect Composite Resin Restorative Systems
Because of major clinical problems clinicians have experienced with direct posterior composite resins, the indirect composite inlay/onlay system was introduced. Superior marginal adaptation, contour and proximal contact can be achieved since the restoration is prepared on a die rather than directly in the cavity preparation[44]. Most clinical studies have demonstrated a dramatic improvement in general clinical performance[45]. Unfortunately, however, the indirect process did not appreciably increase the wear resistance of restorations. While some resin systems may have been somewhat more wear-resistant than others, the differences were not substantial. Post heat-treatment of the composite resins, after light-curing produced appreciable increases in certain mechanical properties[46], [47].
Conclusion
Several varieties of composite restorative materials have been developed but each variety of composite has superior or inferior qualities in one or the other aspects and in their performance. However, research is needed to develop a material with better performance and also research is needed to further evaluate the physical properties and clinical performance of these new restorative materials.
References
1. Mutter J, Naumann J, Sadaghiani C, Walach H, Drasch G. Int. J. Hyg. Environ. Health 2004;207: 391–7.
2. Alsharif SO, Arifin Z, Ishak BM, Arriffin AB. An Overview on Dental Composite Restorative “White Filling”. Annals Inter J Eng 2010; 8: 95-100.
3. Ferracane JL. Current trends in dental composites. Crit Rev Oral Biol Med. 1995; 6: 302–18.
4. Fleisch AF, Sheffield PE, Chinn C, Edelstein BL, Landrigan PJ. Bisphenol A and Related Compounds in Dental Materials. Pediatrics 2010; 126:760–8.
5. Anusavice KJ. Restorative Resins: (In) Philips Science of Dental Materials. 11th ed. Restorative Resins, Elsevier, India 2004; 399-441.
6. Hervás-García A, Martínez-Lozano MA, Cabanes-Vila J, Barjau-Escri¬bano A, Fos-Galve P. Compositeresins. A review of the materials and clinical indications. Med Oral Patol Oral Cir Bucal 2006; 11:215-20.
7. Noort R.V. Introduction to Dental Materials, 2nd ed. Mosby, Elsevier, 2002.
8. Bowen RL, Marjenhoff WA. Dental Composites/Glass Ionomers: The Materials. Adv Dent Res 1992; 6:44-9.
9. Ferracane JL, Greener EH. The effect of resin formulation on the degree of conversion and mechanical properties of dental restorative resins. J Biomed Mater Res 1986; 20:121-31.
10. Asmussen E. Composite restorative resins. Composition versus wall-to-wall polymerization contraction. ActaOdontolScand 1975; 33:337-44.
11. De Gee AJ, Feilzer AJ, Davidson CL. True linear polymerization shrinkage of unfilled resins and composites determined with a linometer, Dent Mater 1993; 9:11-4.
12. Labella R, Lambrechts P, Van Meerbeek B, Vanherle G. Polymerization shrinkage and elasticity of flowable composites and filled adhesives. Dent Mater 1999; 15:128-37.
13. Choi KK, Condon JR, Ferracane JL. The effects of adhesive thickness on polymerization contraction stress of composite, J Dent Res 2000; 79:812-7.
14. Burke FJT, Cheung SW. Restoration longevity and analysis of reasons for the placement and replacement of restorations provided by vocational dental practitioners and their trainers in the United Kingdom. Quintessence Int 1999; 30:234–42.
15. Davidson CL, Feilzer AJ. Polymerization shrinkage and polymerization shrinkage stress in polymer based restoratives. J Dent 1997; 25:435–40.
16. Stansbury JW, Antonucci JM. Evaluation of methylene lactone monomers in dental resins. Dent Mater 1992; 8:270–3.
17. Nie J, Lovell LG, Bowman CN. Synthesis and characterization of N-isopropyl, N-methacryloxyethylmethacrylamide as a possible dental resin. Biomaterials 2001; 22:535–40.
18. Labella R, Davy KWN, Lambrechts P, Van Meerbeek B, Vanherle G. Monomethacrylatecomonomers for dental resins. Eur J Oral Sci 1998; 106:816–24.
19. Moszner N, Zeuner F, Fischer UK, A. de Meijere, Bagutski V. New Bicyclic Cyclopropyl-Acrylates for Dental Composites. Proceeding of the 8th Polymers for Advanced Technologies International Symposium, Budapest, Hungary, September 2005.
20. Bayne SC, Heymann HO, Swift EJ. Update on dental composite restorations. J Am Dent Assoc 1994; 125:687-701.
21. Ferracane JL. In vitro evaluation of composite resins. Structure-property relationships, development of assessment criteria. Trans Acad Dent Mater 1989; 2:6-35.
22. Bouschlicher MR, Cobb DS, Boyer DB. Radiopacity of compomers, flowable and conventional resin composites for posterior restorations. Oper Dent 1999; 24:20-5.
23. Lutz F, Phillips RW. A classification and evaluation of composite resin systems. J Prosthet Dent 1983; 50:480-8.
24. Cramer NB, Stansbury JW, Bowman CN. Recent Advances and Developments in Composite Dental Restorative Materials. J Dent Res 2011; 90:402-16.
25. Willems G, Lambrechts P, Braem M, Celis JP, Vanherle G. A classification of dental composites according to their morphological and mechanical characteristics. Dent Mater 1992; 8:310-9.
26. Swift EJ. Nanocomposites. J EsthetRestor Dent 2005; 17:3-4.
27. Heymann HO, Bayne SC. Current concepts in dentin bonding: focusing on dental adhesion factors. J Am Dent Assoc 1993; 124:26-36.
28. Leinfelder KF. A conservative approach to placing posterior composite restorations. J Am Dent Assoc 1996; 127:743-8.
29. Manhart J, Chen HY, Hickel R. The suitability of packable resin-based composites for posterior restorations. J Am Dent Assoc2001; 132; 639-45.
30. Denehy GE, Vargas M, Cobb DS. Achieving long-term success with class II composite resins. Calif Dent InstContinEduc 1996; 59:27-36.
31. Opdam N, Roeters J, Peters T, Burgersdijk R, Kuijs R. Consistency of resin composites for posterior use. Dent Mater 1996; 12: 350-4.
32. Leinfelder KF, Radz GM, Nash RW. A report on a new condensable composite resin. CompendContinEduc Dent 1998; 19:230-7.
33. Lui JL, Masutani S, Setcos JC, Lutz F, Swartz ML, Phillips RW. Margin quality and microleakage of class II composite resin restorations. J Am Dent Assoc 1987; 114:49-54.
34. McCullock AJ, Smith BG. In vitro studies of cuspal movement produced by adhesive restorative materials. Br Dent J 1986; 161: 405-9.
35. Alani AH, Toh CG. Detection of microleakage around dental restorations: a review. Oper Dent 1997; 22:173-85.
36. Wilson E, Mandradjieff M, Brindock T. Controversies in posterior composite resin restorations. Dent Clin North Am 1990; 34: 27-44.
37. Sakaguchi RL, Douglas WH, Peters MC. Curing light performance and polymerization of composite restorative materials. J Dent 1992; 20:183-8.
38. Christensen GJ. Conservative posterior tooth restorations. J Esthet Dent 1993; 5: 154-60.
39. Leinfelder KF, Prasad A. A new condensable composite for the restoration of posterior teeth. Dent Today 1998; 17:112-6.
40. Hickel R, Dasch W, Janda R, Tyas M, Anusavice K. New direct restorative materials: FDI Commission Project. Int Dent J 1998; 48:3-16.
41. Wolter H, Storch W, Ott H. New inorganic/organic copolymers (ORMOCERs) for dental applications. Mat Res SocSympProc 1994; 346:143-9.
42. Sivakumar A, Valiathan A. Dental Ceramics and Ormocer Technology – Navigating the future, Trends BiomaterArtif Organs 2006; 20: 40-3.
43. Rama Krishna Alla. Restorative Materials: Composite Resins, (In) Dental Materials Science, Jaypee Brothers Medical Publishers (P) Ltd, New Delhi, India 2013; 130-48.
44. Peumans M, Van Meerbeek B, Ascherickx K, Simon S, Abe Y, Lambrechts P, Vanherle G. Do condensable composites help to achieve better proximal contacts? Dent Mater 2001; 17:533-41.
45. Leinfelder KF. New developments in resin restorative systems. J Am Dent Assoc 1997; 128: 573-81.
46. Wendt SL. The effect of heat used as a secondary cure upon the physical properties of three composite resins. I. Diametral tensile strength, compressive strength and marginal dimensional stability. Quintessence Int 1987; 18:265-71.
47. Wendt SL. The effect of heat used as a secondary cure upon the physical properties of three composite resins. II. Wear, hardness and color stability. Quintessence Int. 1987; 18:351-6.
|