Introduction
Nanotechnology is the creation and utilization of materials, devices and systems through the control of matter on the nanometer-length scale, that is, at the level of atoms, molecules and supramolecular structures. Nanobiotechnology is already starting to show the promise of an impact on health care. [1]
Nanomedicine is defined as the application of nanobiotechnology to medicine and is based on the use of nanoscale materials and devices for diagnosis and drug delivery as well as for the development of advanced pharmaceuticals referred to as nanopharmaceuticals. [1]
Nanobiotechnology is also being applied to refine surgery from microsurgery to nanosurgery. Examples include the construction of nanoscale robots, nanobots, for navigating the human body to detect as well as treat various diseases, and cell surgery using nanodevices and nanolasers. During the past few years, considerable progress has been made in the application of nanobiotechnology in cancer, that is, nano-oncology, which is recently the most important chapter of nanomedicine. [1]
Nanobiotechnology plays an important role in the discovery of biomarkers of cancer. Several drugs in development for cancer are based on nanobiotechnology, and a few of these are already approved. Nanobiotechnology- based devices are in development as aids to cancer surgery. Finally, nanobiotechnology is playing an important role in personalized therapy for cancer. [1]
This review focuses on the current concepts and the application of nanotechnology in by means of nano-systems in cancer diagnosis and treatment.
Historical Background
Nanotechnology deals with structures that range from 1 to l00nm- about the size of a virus and 'Nano' derives its name from Greek word for "dwarf".[2] The conceptual underpinnings of nanotechnologies were first laid out in 1959 by the physicist Richard Feynman in his lecture, "There's plenty of room at the bottom". The term nanotechnology was not used until 1974, when Norio Taniguchi, a researcher at the University of Tokyo, used it to refer to the ability to engineer materials precisely at the nanometer level. The primary driving force for miniaturization at that time came from the electronics industry, which aimed to develop tools to create smaller electronic devices on silicon chips. [3]
According to James R Baker Jr "Nanotechnology allows us to make materials that are thousands of times smaller than the smallest cell in the body". Because these materials are so small, they can easily get inside cells and change how they work. [4]
Development Of Nanotechnology
Mihail (Mike) Roco of the U.S. National Nanotechnology Initiative has described four generations of nanotechnology development. The 1st generation, as Roco depicts it, is that of passive nanostructures, materials designed to perform one task. The 2nd generation introduces active nanostructures for multitasking; for example, actuators, drug delivery devices, and sensors. The 3rd generation features nano-systems with thousands of interacting components. A few years later, the first integrated nano-systems, functioning (according to Roco) much like a mammalian cell with hierarchical systems within systems, are expected to be developed. [5]
Some experts may still insist that nanotechnology can refer to measurement or visualization at the scale of 1-100 nanometers, but a consensus seems to be forming around the idea that control and restructuring of matter at the nano-scale is a necessary element. As work progresses through the four generations of nanotechnology leading up to molecular nano-systems, which will include molecular manufacturing, we think it will become increasingly obvious that "engineering of functional systems at the molecular scale" is what nanotech is really all about. [5]
Nanosystem's in cancer diagnostics
Nano-biotechnology offers a novel set of tools for the detection of cancer and contributes to early detection of cancer. [6]
Types of Nanosystem's
These include as tabulated in [Table 1].
Application Of Nanotechnology In Oncology
Nanotechnology may have an impact on the key challenges in cancer diagnosis and therapy. Diagnosing, treating, and tracking the progress of therapy for each type of cancer has long been a dream among oncologists, and one that has grown closer to parallel revolutions in genomics, proteomics and cell bio1ogy. Nanotechnology's greatest advantage over conventional therapies may be the ability to combine more than one function. Recently, there is a lot of research going on to design novel 'Nanodevices' capable of detecting cancer at its earliest stages, pinpointing its location within the human body and delivering chemotherapeutic drugs against malignant cells. The major areas in which nanomedicine is being developed in oncology involve [2]:
Early detection of tumor (developing "smart" collection platforms for simultaneous analysis of cancer-associated markers and designing contrast agents that improve the resolution of tumor area comparing with the nearby normal tissues), and
Cancer treatment (creating nanodevices that can release chemotherapeutic agents)[2]:
1. Detection of tumor
Early detection of tumor will greatly increase survival rates with the reasonable assumption that an in situ tumor will be easier to eradicate than one that has metastasized. Nanodevices and especially nanowires can detect cancer-related molecules, contributing to the early diagnosis of tumor. Nanowires having the unique properties of selectivity and specificity can be designed to sense molecular markers of malignant cells. They are laid down across a microfluidic channel and they allow cells or particles to flow through it. Nanowires can be coated with a probe such as an antibody or oligonucleotide. Proteins that bind to the antibody will change the nanowire's electrical conductance and this can be measured by a detector. As a result, proteins produced by cancer cells can be detected and earlier diagnosis of tumor can be achieved. [14]
Nanoparticle contrast agents are being developed for tumor detection purposes. Labeled and non-labeled nanoparticles are already being tested as imaging agents in diagnostic procedures such as nuclear magnetic resonance imaging.[15] There are two main groups of nanoparticles: 1) superparamagnetic iron oxides whose size is greater than 50 nm, 2) ultrasmall superparamagnetic iron oxides which are smaller than 50nm.[16] Moreover, Quantum dots can be used to measure levels of cancer markers such as breast cancer marker Her-2, actin, microfibril proteins and nuclear antigens.[17]
Nanotechnology for detection of cancer biomarkers
Any specific molecular alteration of a cell on the DNA, RNA, metabolite or protein level may be referred to as a molecular biomarker. From a practical point of view, the biomarker would specifically and sensitively reflect a disease state and could be used for diagnosis as well as for disease monitoring during and following therapy. [18] Currently, available molecular diagnostic technologies have been used to detect biomarkers of various diseases such as cancer. Nanotechnology has further refined the detection of biomarkers. The physicochemical characteristics and high surface areas of nanoparticles make them ideal candidates for developing platforms for harvesting biomarkers. Some biomarkers also form the basis of innovative molecular diagnostic tests. A magnetic nanosensor technology is up to 1,000 times more sensitive than any technology now in clinical use, can detect biomarker proteins over a range of concentrations three times greater than any existing method and is accurate regardless of which bodily fluid is being analyzed.[19] The nanosensor chip also can search for up to 64 different proteins simultaneously and has been shown to be effective in early detection of tumors in mice, suggesting that it may open the door to significantly earlier detection of even the most elusive cancers in humans.[1]
Investigating the potential for capturing circulating tumor cells
A method has been described for magnetically capturing circulating tumor cells in the bloodstream of mice followed by rapid photoacoustic detection. [20] Magnetic nanoparticles are functionalized to target a receptor commonly found in breast cancer cells, which bind and capture circulating tumor cells under a magnet. The approach of integrating in-vivo multiplex targeting, magnetic enrichment, signal amplification and multicolor recognition, enables circulating tumor cells to be concentrated from a large volume in the vessels of tumor-bearing mice and has potential applications for the early diagnosis of cancer and the prevention of metastasis in humans. [1]
Imaging applications of Nano-biotechnology in cancer
Highly lymphotropic superparamagnetic iron oxide nanoparticles (SPIONs), measuring 2 to 3 nm on average have been used in conjunction with high-resolution MRI to reveal small and otherwise undetectable lymph node metastases.[21] Quantum dots are attractive as optical imaging agents owing to their high brightness and photo- and biostability. Bioluminescence resonance energy transfer Quantum dots can improve the signal-to-background ratio for real-time imaging largely by suppressing background signal. [22]
2. Tumour treatment
Frequent challenges encountered by
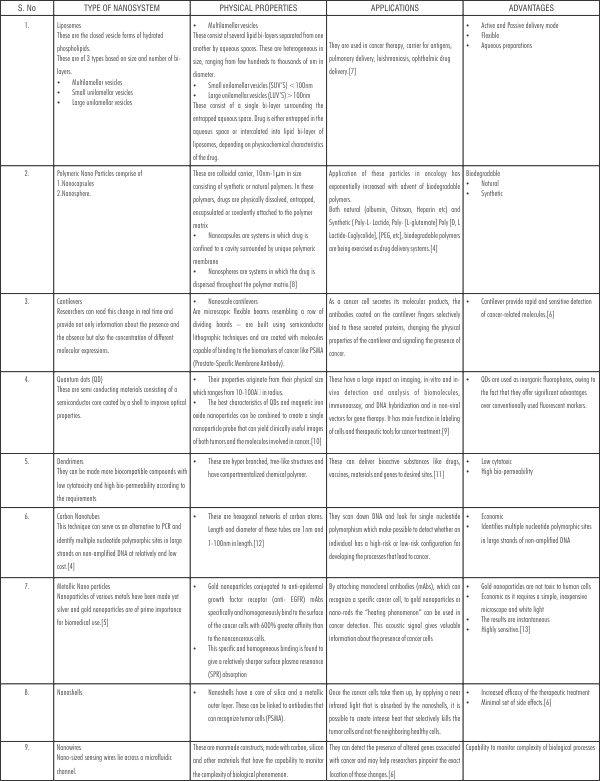 | Table 1
 |
current cancer therapies include nonspecific distribution of anti-tumour agent, inadequate drug concentrations reaching the tumor, and limited ability to monitor therapeutic responses. Poor drug delivering to the target site leads to significant complication, such as multidrug resistance. [23] Current NT promises solutions to several of the current obstacles facing cancer therapies. Nanoparticles have size of 5nm to 200nm, allowing their unique interaction with biological systems at the molecular level. As a result of their materials composition, nanoparticles are capable of self-assembly and maintaining stability and specificity which are crucial to drug encapsulation and biocompatibility. [4] Nanoparticles can consist of a number of materials, including polymers, metals and ceramics. Many types of nanoparticles are under various stages of development as drug delivery systems, including liposomes and lipid based carriers (such as lipid emulsions and lipid -drug conjugates, polymer microspheres, micelles and various ligand - targeted products (such as immunoconjugates). [2] As an example, a nanoparticle-based drug called "Abraxane", consisting of paclitaxel conjunctive to protein albumin particles, was approved by the Food and Drug Administration for breast cancer treatment a year ago.[24] It is worthwhile to mention that selective delivery and targeting of nanoparticles to tumors may overcome the problem of toxicity and may increase the effectiveness of drug delivery. The barriers involving this procedure and that should be under consideration are a variety of physical and anatomical characteristics of solid tumors, such as the necrotic core with the surrounding hypoxic area, the elevated local temperature and the interstitial liquid pressure. [25], [26], [27]
Several approaches have been used to target nanoparticles to tumor associated antigens, including direct conjugation of nanoparticles to monoclonal antibodies, modified plasma proteins or viral vectors. Recent progress has been made with targeted viral vectors for gene therapy applications. [28]
In addition to this, laser-induced thermal effects around nanoparticles attached to specific targets have recently been used for the treatment of cancer. The basic concept for this application of Nanotechnology is the fact that nanoparticles of different properties (magnetic, optical etc.), due to their size, can be delivered more easily to target cells than can larger particles, via conjugation with antibodies, conjugation to viruses and physiological transportation.[29]
After reaching target cells, these nanoparticles are then self-assembled into larger nanoclusters within cells. Afterwards, these nanoclusters can be activated by laser irradiation, microwaves or magnetic fields, depending on the nanoparticles synthesis. By this process and its photothermal effects, destruction of cancer can be achieved. More specifically, the nanoshell-assisted photo-thermal therapy (NAPT) is a non-invasive procedure for selective photo-thermal tumor destruction. It is based on nanoshells that absorb light in the near infrared (NIR) region, which is the wavelength that optimally penetrates tissues. The metal shell converts the absorbed light into heat with great efficacy. Further specificity can be engineered by attaching antigens on the nanoshells which are specifically recognized by the cancer cells. By supplying a light in NIR from a laser, the particles produce heat, which destroy the tumor. [30]
In-vivo, Raoul Kopelman et al; have recently created three-component nanoparticles that target, image and destroy tumors in the brains of rats. The particles consist of an iron oxide core that serves as a magnetic resonance imaging (MRI) contrast agent. Attached to them are copies of a cancer-targeting peptide called F3, as well as a light-absorbing compound called photofrin that kills cells when hit with red light. When Kopelman's team used their combination particles to treat rats previously injected with cancer cells inside their brains, animals receiving the combination particles survived more than twice as long as control animals receiving the non-targeted photofrin compound.[31]
Ultrasonic tumor imaging and targeted chemotherapy by nanobubbles
Drug delivery in polymeric micelles combined with tumor irradiation by ultrasound results in effective drug targeting, but this technique requires prior tumor imaging.[32] Multifunctional nanoparticles that are tumor-targeted drug carriers, long-lasting ultrasound contrast agents and enhancers of ultrasound-mediated drug delivery have been developed and deserve further exploration as cancer therapeutics.[1]
Nanoparticle-based thermal ablation of cancer
Several forms of energy have been used for the destruction of tumor cells that cannot be reached for conventional surgical excision. Thermal ablation therapy is the most promising of these methods but is limited by incomplete tumor destruction and damage to adjacent normal tissues. Use of nanoparticles has refined noninvasive thermal ablation of tumors, and several nanomaterials have been used for this purpose. These include gold nanomaterials, iron nanoparticles, magnetic nanoparticles and carbon nanotubes. Heating of the particles can be induced by magnets, lasers, ultrasound, photodynamic therapy and low-power X-rays. [33]
Laser-induced thermal destruction of cancer using nanoparticles
Single-walled carbon nanotubes (SWCNTs) show strong optical absorbance 700- to 1,100-nm NIR laser impulses. SWCNTs emit heat when they absorb energy from NIR light. Tissue is relatively transparent to NIR, which suggests that targeting SWCNTs to tumor cells, followed by noninvasive exposure to NIR light, will ablate tumors within the range of NIR. One study has demonstrated the specific binding of mAb-coupled SWCNTs to tumor cells in vitro, followed by their highly specific ablation with NIR light. [34] Only the specifically targeted cells were killed after exposure to NIR light. Selective cancer cell destruction can be achieved by functionalization of SWCNTs with a folate moiety, selective internalization of carbon nanotubes inside cells labeled with folate receptor tumor biomarkers, and NIR-triggered cell death, without harming receptor-free normal cells.[1]
Role Of Nanotechnology In Personalized Therapy Of Cancer
Personalized medicine simply means the prescription of specific therapeutics best suited for an individual. Personalized management is usually based on pharmacogenetic, pharmacogenomic, pharmacoproteomic and pharmacometabolic information, but other individual variations in patients and environmental factors are also taken into consideration. [35]
In cancer cases, the variation in behavior of cancer of the same histological type from one patient to another is also taken into consideration. Personalization of cancer therapies is based on a better understanding of the disease at the molecular level, and nanotechnology will play an important role in this area. [36] With so many nanotechnologies available for drug delivery, it is recommended that computational mathematical tools be used to predict, which parameter's to be used for a multistage drug-delivery strategy. This would enable efficient localized delivery of chemotherapeutic drugs and lead to significant improvements in therapy efficacy as well as reduced systemic toxicity.[37]Such an approach can be optimized for personalized oncology.
Advantages of Nanotechnology
Imaging agents and diagnostics that will allow clinicians to detect cancer in its earliest stages
Multifunctional, targeted devices capable of bypassing biological barrier to deliver multiple therapeutic agents directly to cancer cells
Agents that can monitor predictive molecular changes and prevent precancerous cells from becoming malignant
Novel methods to manage the symptoms of cancer that adversely impact quality of life
Research tools that will enable rapid identification of new targets for clinical development and predict drug resistance.[6]
Nanotechnology as a risk to human health
Although the benefits of nanotechnology are widely publicized, discussion of the potential effects of their widespread use in consumer and industrial products is just beginning. Both pioneers of nanotechnology and its opponents are finding it extremely hard to argue their case because of the limited information available to support one side or the other. Given the rapid rate of development in this area and the amount of publicity it is attracting, it is not surprising that concerns should have been raised relating to the safety of nanomaterials in a variety of products. [38], [39] Although some concerns may be ill-founded, it remains true that the toxicology of many nanomaterials has not yet been fully evaluated.
It has been shown that nanomaterials can enter the human body through several ports. Accidental or involuntary contact during production or use is most likely to occur via the lungs, from which a rapid translocation is possible to other vital organs through the bloodstream.
On the cellular level, an ability to act as a gene vector has been demonstrated for nanoparticles.
Carbon black nanoparticles have been implicated in interfering with cell signaling. There is work that demonstrates uses of DNA for the size separation of carbon nanotubes. The DNA strand just wraps around it if the tube diameter is right. Though excellent for the purposes of separation, this tendency raises some concerns over the consequences of carbon nanotubes entering the human body.[40], [41]
Conclusions
Nanotechnology in modern medicine and nanomedicine is in infancy, having the potential to change medical research dramatically in the 21st century.
Nanomedical devices can be applied for analytical, imaging, detection, diagnostic and therapeutic purposes and procedures, such as targeting cancer, drug delivery, improving cell-material interactions, scaffolds for tissue engineering, and gene delivery systems, and provide innovative opportunities in the fight against incurable diseases.
There has been a huge progress on understanding the function of biological structures of nanotechnology tools and techniques and their interaction and integration with several non-living systems, but there are still open issues to be answered, mainly related to biocompatibility of the materials and devices which are introduced into the body.
Many promising novel nanoparticles and nanodevices are expected to be used, with an enormous positive impact on human health.
The vision is to improve health by enhancing the efficacy and safety of nanosystems and nanodevices.
In the coming years, nanotechnology will play a key role in the medicine of tomorrow providing revolutionary opportunities for early disease detection, diagnostic and therapeutic procedures to improve health and enhance human physical abilities, and thus enabling precise and effective therapy tailored to the mankind.
References
1. Jain KK. Advances in the field of Nanooncology. BMC Med 2010; 8:83.
2. Logothetidis S. Nanotechnology in medicine. The medicine of tomorrow and nanomedicine. Hippokratia 2006; 10:7-21.
3. Sahoo SK, Parveen S, Panda JJ. The present and future of nanotechnology in human health care. Nanomedicine 2007 Mar; 3:20-31.
4. Ravindran R. Nanotechnology in cancer diagnosis and treatment: An overview. Oral and Maxillofacial Pathology Journal 2011; 2:101-06.
5. Rangaswamy M. Nanotechnology A Review. J of applied pharmaceutical science 2001; 1:8-16.
6. Satya KS, Shrinivasa BP. Nanotechnology: A new approach in cancer therapy. Pharma times April 2006; 38:17-20.
7. Jain S, Jain NK. Liposomes as drug carrier. In Jain NK (ed). Text book of controlled and normal drug delivery, 2nd edition. New Delhi, CBS publisher, 2002; 304-52.
8. Maincent P, Marchal-Heussler I, Sirbat D, Thouvenotp, Hoffman M, Vallet JA. Proceedings of international symposia; controlled released bioactive materials 1992; 18:226.
9. Bailey RE, Smith AM, Nie S. Quantum dots in biology and medicine. Physica E 2004; 25:1-12.
10. Choi JS, Jun YW, Yeon SI, Kim HC, Shin JS, Cheon J. Biocompatible heterostructured nanoparticle for muti-modal biological detection. J Am Chem Soc 2006; 128:15982-3.
11. Kreuter J, Speiser PP. In vitro studies of poly (methyl methacrylate) adjuvants. J of Pharm Sci 1976; 65:1624-7.
12. Sinha N, Yeow JT. Carbon nanotubes for biomedical applications. IEEE Trans Nanobioscience 2005;4(2):180-95 .
13. El Sayed IH, Huang X, L Sayed M. Selective laser photo thermal therapy of epithelial carcinoma using anti EGFR antibody conjugated gold nanoparticle. Cancer Lett 2006; 239:129-35.
14. Zadonella C. The tiny tool kit. Nature 2003; 433:10-12.
15. Brigger I, Dubernet C, Couvreur P. Nanoparticles in cancer therapy and diagnosis. Adv Drug Deliv Rev 2002; 54:631-51.
16. Bonnemain. Super paramagnetic agents in MRI. Physiochemical characteristics and clinical applications-A review. J Drug Target 1998; 6:167-74.
17. Kawasaki ES, Player TA. "Nanotechnology, nanomedicine and the development of new, effective therapies of cancer". Nanomedicine 2005; 1:101-9.
18. Jain KK. Biomarkers and Personalized Medicine. In, Jain KK(ed). A handbook of biomarkers, 1st edition. Newyork, Spinger, 2010; 433-42.
19. Gaster RS, Hall DA, Nielsen CH, Osterfeld SJ, Yu H, Mach KE et al. Matrix-insensitive protein assays push the limits of biosensors in medicine. Nat Med 2009; 15:1377-82.
20. Galanzha EL, Shashkov EV, Kelly T, Kim JW, Yang L, Zharov VP. In-vivo magnetic enrichment and multiplex photoacoustic detection of circulating tumor cells. Nat Nanotechnol 2009; 4: 855-60.
21. Ross RW, Zietman AL, Xie W, Coen JJ, Dahl DM, Shipley WU et al. Lymphotropic nanoparticle enhanced MRI identifies occult lymph node metastasis in prostate cancer patients prior to salvage radiation therapy. Clin Imaging 2009; 33:301-05.
22. Kosaka N, McCann TE, Mitsunaga M, Choyke PL, Kobayashi H. Real time optical imaging using quantum dot and related nanocrystals. Nanomedicine 2010; 5:765-76.
23. Wang X, Yang L, Chen Z, Shin DM. Application of nanotechnology in cancer therapy and imaging. CA Cancer J Clin 2008; 58:97-110.
24. Tachung C, Yie PE, Wie C. "Nanomedicine in cancer medicine." Nanotechnology 2005; 1:191-2.
25. Valdimir P, Zharov DSC, Jin-woo Kim, David T Curiel, Maaike Everts. "Self-assembling nano clusters in living system: application for integrated photothermal nanodiagnostics and nano therapy". Nanomedicine 2005; 1:326-45.
26. Zharov V, Galitovsky V, Viegas M. Photothermal detection of local thermal effects during selective nanophoto thermolysis. Appl Phys Lett 2003; 83:448-97.
27. Zharov VP, Galitovsky V, Viegas M. Photothermal guidance of selective photothermolysis with nanoparticles. Proceedings of SPIE 2004; 5319:291-300.
28. Everts M, Curiel DT. Transductional targeting of adenoviral cancer gene therapy. Curr Gene Ther 2004; 4:337-46.
29. O' Neil DP, Hirsch LR, Halas NJ, Payne JD, West JL. Photothermal tumor ablation in mice using near infrared absorbing nanoparticles. Cancer Lett 2004; 209:171-76.
30. Bradbury J. Nanocell destruction of inoperable tumors. Lancet oncology 2003; 4:711.
31. Service RF. Nanotechnology takes aim at cancer. Science 2005; 18:1132-4.
32. Rapoport N, Gao Z, Kennedy A. Multifunctional nanoparticle for combining ultrasonic tumor imaging and targeted chemotheraphy. J Natl Cancer Inst 2007;99:1095-106.
33. Jain KK. Nanoparticles for Targeting Tumors. In, Jain KK (ed). Handbook of Nanomedicine. Humana, Springer Totowa NJ 2008;204-05.
34. Chakravarthy P, Marches R, Zimmerman NS, Swafford AD, Bajaj P, Musselman IH et al. Thermal ablation of tumor cells with antibody-functionalised single-walled carbon nanotubes. Proc Natl Acad Sci USA 2008; 105:8697-702.
35. Jain KK. Future of cancer therapies. In, Jain KK(ed). Textbook of personalized medicine. Newyork, Springer 2009;250-54.
36. Jain KK. Role of nanotechnology in developing personalized medicine for cancer. Technol Cancer Res Trait 2005;4:407-16.
37. Sakamoto J, Annapragada A, Decuzzi P, Ferrari M. Antibiological barrier nanovector technology for cancer aplications. Expert Opin Drug Deliv 2007;4: 359-69.
38. Matsudai M, Hunt G. Nanotechnology and public health. Nippon Koshu Eisei Zasshi 2005;52:923-7.
39. Hoet PH, Bruske-Hohlfeld I, Salata OV. Nanoparticles-known and unknown health risks. J Nanobiotechnology 2004;8:12.
40. Williams D. Nanotechnology: a new look. Med Device Technol 2004;15:9-10.
41. Williams D. The risk of nanotechnology. Med Device Technol 2005;16:9-10. |